Drug Discovery Evolves: Patient-Focused Approaches and AI Integration Lead the Way
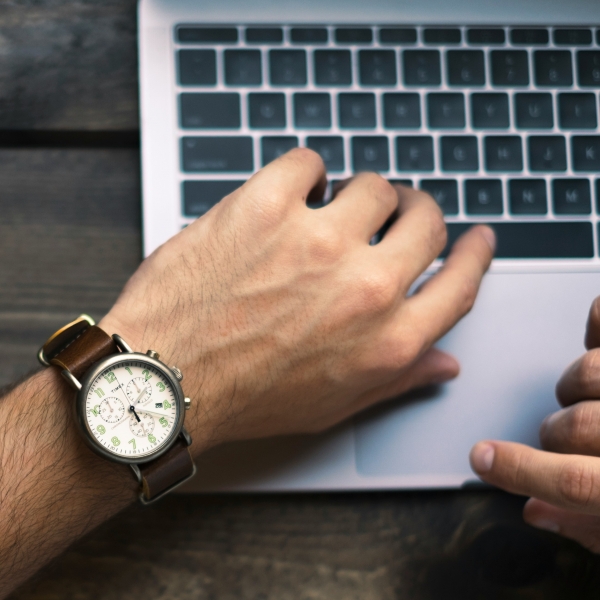
By: Toni Shears
Imagine that your doctor has diagnosed you with irritable bowel syndrome but is not sure what’s causing it or why you’re not responding to treatment. Working from a tiny tissue sample, Jonathan Sexton, PhD, can basically recreate your specific disease in the lab and rapidly test hundreds of drugs to see which might bring you relief.
Sexton, an Assistant Professor of Medicinal Chemistry in the College of Pharmacy, brings cutting-edge drug discovery technology to clinical care at Michigan Medicine, helping to develop more effective and personalized treatments.
“Part of what we do in my lab is precision patient modeling. That means we get cells from patients with a disease. Using tissue engineering and AI-based screening together, we attempt to correct their phenotypes and discover the ideal treatment for each patient,” he says.
For example, in a new clinical research program, “we obtained biopsy tissue from patients with inflammatory bowel disease, then developed a cell model of that specific patient and their disease,” he explains. “That showed us that the patient had inflammation and intestinal barrier permeability, or a leaky gut, which propagates the inflammatory response and exacerbates the disease state.”
From there, his team can expose their patient-specific cell models to drugs or dietary supplements and analyze the cellular response. “This way, we can rapidly test many different kinds of interventional strategies to see if we can repair that defect.”
Picturing Evidence of Cures
To this high-tech combination of tissue engineering and drug discovery, Sexton’s lab brings an added focus. His team specializes in high-tech biological imaging paired with high-throughput analysis that spots positive reactions at the cellular level.
“We have a lot of drugs to test against our engineered tissue, so we’re screening in 96-well plates and 384-well plates to do massive parallel experimentation. Then we need to see the tissue to assess its health and compare responses,” he explains. “We do that by multiplex biological imaging,”
With a $1.5 NIH grant, Sexton’s lab installed an automated epifluorescence microscope to image samples and a robot to automate the process of imaging and analyzing all those samples. The robot arrived in late September and is expected to be operational in January 2025.
“The evidence of disease or health is revealed in the images of their tissue, and we can take more than a million pictures of cells daily,” Sexton explains. “We can't analyze all of them by eye, so we use machine vision and artificial intelligence technologies to inspect those images and find images where the drug improved the condition or corrected the disease.”
Engineering a Liver in the Lab
The overarching focus of Sexton’s tissue engineering work is to build better, more physiologically relevant models of cellular and organ function to enhance drug discovery. He focuses on modeling liver cells because the liver does the heavy lifting of metabolizing drugs — and the liver is the primary site for drug toxicity.
Traditional methods of growing cells in the lab to test drugs have serious limitations. Researchers often started with cancer cells because they grow fast and can be cultivated easily and replicated for years. But when working with cancer cells from the liver, over time, those cultured cells lose the liver’s unique functionality.
“At some point, liver cancer cells no longer metabolize drugs, secret albumin, and do the other important things that maintain your physiology. They've been cultured in vitro for so long that they really just aren't liver anymore. They are not very good models for human disease.”
Sexton’s team is also moving beyond the hepatocyte, the workhorse liver cell that has been the root of most research. “We are engineering liver tissue with many different cell types. We’re trying to mimic the entire liver with all its complexity,” he explains.
He doesn’t even need to start with a tissue sample. “We take a simple, standard 10-ml tube of blood collected when the patient is in the clinic. From there, we extract white blood cells and reprogram them into stem cells. Then, from the stem cells, we can actually make a piece of their liver tissue, so now we have a representation of that unique individual in a dish. We can engineer a piece of their liver, which we call a human liver organoid. With that, we can see how their liver performs under certain scenarios.”
These models replicate normal functions and disease states much more reliably than old methods. Crucially, they also allow researchers to investigate an individual’s particular disease or idiosyncratic response to a drug — and responses across different populations. “We can get blood from anybody we want, whatever demographic we want, whatever age, whatever sex, and model that,” Sexton says. “That's really important to us.” It opens the door to testing drugs in specific populations and understanding idiosyncratic responses to drugs.
Probing Drug Toxicity and Failure
As the key player in drug metabolism, the liver is also a major reason why new drugs in development fail. Compounds that cause toxic reactions never get beyond initial safety tests. But even those that are found to be safe for most patients will cause liver toxicity in a few — and that may be enough to keep the drug off the market.
Sexton, who holds a joint appointment in Internal Medicine at the U-M Medical School, uses his advanced tissue modeling techniques to better understand why some patients react dramatically and differently to a drug.
The common antibiotic Augmentin “is our worst offender,” Sexton says. “Every once in a while, for one patient in a few thousand, it will cause such severe liver injury that is life-threatening. They experience something like an autoimmune attack on the liver. It's really strange.”
When potential new drugs because of liver injury, “they fail when they are close to the finish line, in Phase III clinical trials or even after a drug hits the market,” Sexton says. After investing years and millions of dollars in a promising compound, a drug that may bring great benefits to most patients will be recalled, black-boxed or not prescribed because it’s dangerous for a few. “We’re trying to find the underlying mechanism of this liver reaction to learn how to avoid this in the future,” he says.
Sexton’s team finds patients who’ve experienced this toxicity through the Michigan Hepatotoxicity Clinical Network, a decades-long NIH-funded effort to track and study liver injury run by Professor of Internal Medicine Robert Fontana, Sexton’s colleague at Michigan Medicine.
“Just in the last year we've, we've made stem cell models out of 18 patients who have experienced drug-induced injury. Our goal is to figure out why these people manifested this horrible effect from common drugs that many people take. We’re trying to learn how to avoid this and make drugs safer for people.”
Visualizing Cures for Killer Diseases
Sexton’s more realistic tissue models, combined with powerful machine-learning and imaging techniques, are yielding clues to treatments and potential cures for diseases like diabetes, obesity, metabolic syndrome, and other conditions that affect millions.
For example, one in four Americans has nonalcoholic fatty liver disease, also known as metabolic-associated steatotic liver disease (MASLD). It can progress to liver injury and failure and is linked to liver cancer, yet there are no drugs to treat it. Using robotic methods to speed the process, Sexton’s team has tested thousands of compounds against their engineered liver tissue to find some that can nudge liver cells to shed fat.
“We have found many compounds that can do that. We can see under the microscope that cells are shedding their fat and look healthy,” Sexton says. “We feel these compounds have strong clinical translational potential, so we're working on how to develop them into a drug that can prevent or cure MASLD.
“There is one compound we’re very excited about because it’s hitting a novel target. With this substance, we’ve successfully reversed fatty liver disease in animal models in 12 days.”
He is seeing equally dramatic results with diabetes. With colleague Martin Clasby, an associate research scientist in Medicinal Chemistry at the College of Pharmacy, he is isolating compounds that can spur pancreatic cells to regenerate precious insulin-producing beta cells. Pancreatic cells are harder to engineer, so they start with donated cadaveric organs, isolate the cells, grow them in culture, and treat them with drugs to see if they will regenerate. “We’ve successfully done that. It’s exciting,” Sexton reports.
Sexton's lab has also made inroads on infectious diseases. They leveraged imaging capabilities to actually visualize viral infections in human cells, providing unprecedented insights into viral behavior. “This work started with COVID-19. We’ve moved on to study many more viruses,” Sexton says. They identified antiviral drugs that have gone forward to clinical trials. Some have been approved. “We’re working on new antivirals where we’ve made some significant discoveries and are in licensing talks with a few pharmaceutical companies now to see if we can advance these potential drugs for viral diseases,” he adds.
“It’s a great time to be doing drug discovery, especially in the area of personalized medicine,” Sexton says. “We're experiencing a confluence of transformative technologies for drug discovery. One is precision patient modeling through stem cells and tissue engineering. Another is artificial intelligence. Combined with all the modern molecular biology tools, these methods allow us to make really, really rapid progress.