LABORATORY
Sun Laboratory
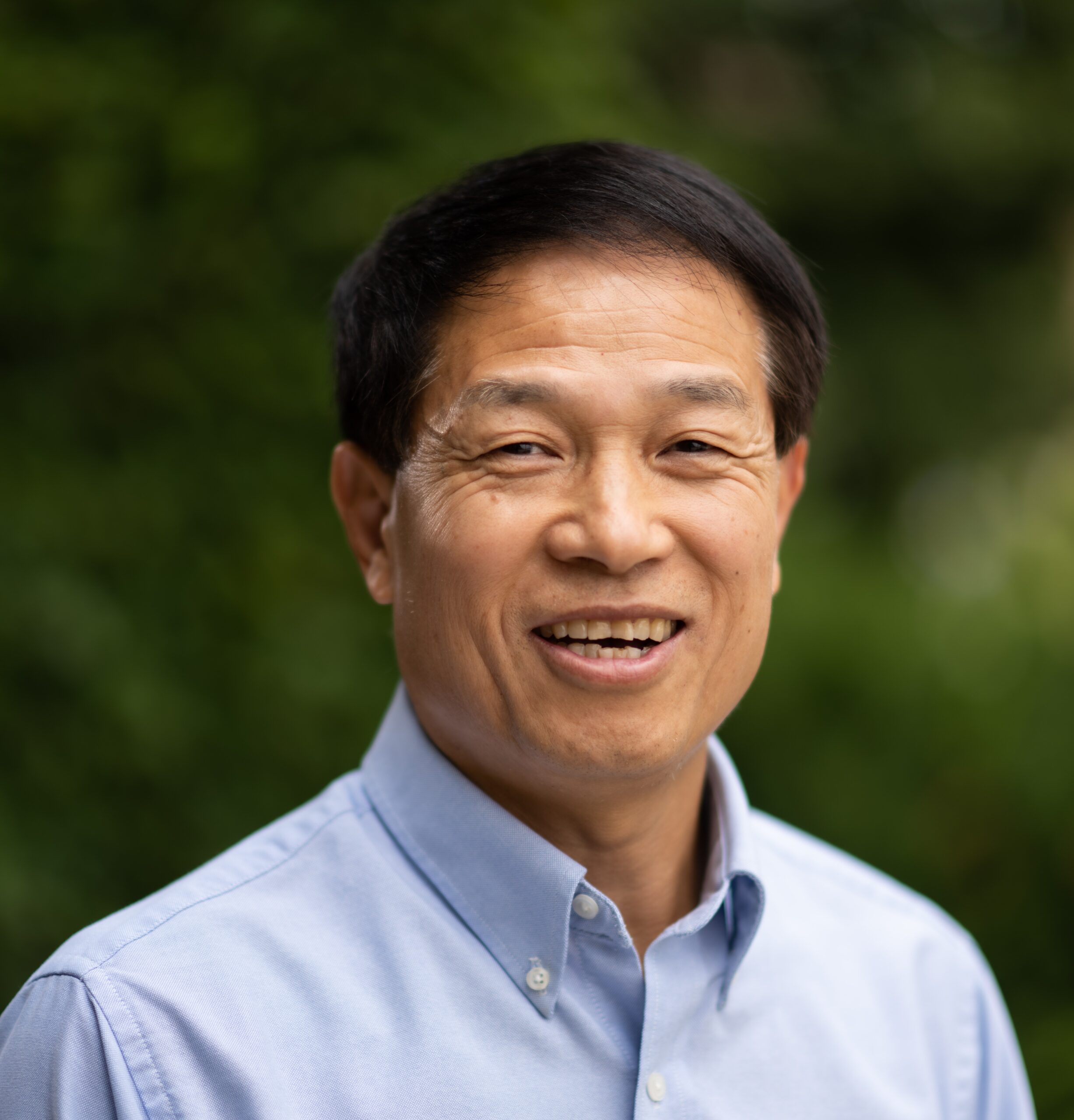
Duxin Sun, PhD
Dr. Duxin Sun is the Associate Dean for Research, the Charles Walgreen Jr. Professor of Pharmacy and Pharmaceutical Sciences in the College of Pharmacy at the University of Michigan. He serves as the Director of the Pharmacokinetics (PK) Core. Dr. Sun also has a joint appointment in the Chemical Biology program, the Interdisciplinary Medicinal Chemistry program, and University of Michigan’s Comprehensive Cancer Center.
Dr. Sun’s research interests focus on drug development, cancer nanomedicine, cancer vaccine, and pharmacokinetics. Dr. Sun established the STAR system (Structure-Tissue/Cell Selectivity-Activity Relationship) to enhance drug development success rate by addressing the 90% failuar rate. He designed albumin based nanomedicines to enhance clinical efficacy of immuno-oncology drugs by targeting immune cells in the lymphatic system and tumors. He also developed SARS-CoV-2 B epitope-guided neoantigen peptide or mRNA vaccines to enhance their efficacy by activating CD4/CD8 T cell immunity through B cell-mediated antigen presentation.
Dr. Sun earned his BS in Pharmacy, MS in Pharmacology, and PhD in Pharmaceutical Sciences, and has also received training in Molecular Biology as a visiting scientist. With research experience in both academia and the pharmaceutical industry, Dr. Sun has published over 280 papers, and has mentored 40 PhD students and 75 postdoctoral fellows/visiting scientists. Dr. Sun is an elected Fellow of both the American Association for the Advancement of Science (AAAS) and the American Association of Pharmaceutical Scientists (AAPS). He has served on the FDA Pharmaceutical Science and Clinical Pharmacology Advisory Committee and participated in study sections for the NIH and FDA.
Dr. Sun’s administrative Specialist is Erika Zucal – [email protected].
Lab and Research Overview
1. Why 90% drug development fails and how to improve it?
This project aims to enhance drug development success and efficiency through the STAR-guided drug design of dual targeting PI3Kγ/Sting, PI3Kγ inhibitors, and STING agonists/antagonists, JAK inhibitors for immunotherapy of cancer or autoimmune disease.
The 90% failure rate of drug development has not improved over the past 40 years despite significant improvement at each step of cancer drug development. It is impractical to add more testing/criteria to the already lengthy and costly drug development process, which takes 10-15 years and costs $1-2 billion per approved drug. The current strategies to the problem, including AI-driven solutions, may fall into the “survivorship bias” trap, which overly focuses on many unimportant peripheral problems but overlooks the root causes of the failures.
We propose the STAR-guided drug design system (structure-tissue/cell selectivity-activity-relationship) to enhance both success rate and efficiency. This system addresses the three overlooked interdependent factors: (a) On-/off-target driven potency/specificity (PS) and on/off-target tissue/cell selectivity (PS) in disease tissues at clinically relevant doses that determine clinical efficacy. (b) on-/off-target-driven PS and TS in normal organs at clinically relevant doses that influence adverse effects (on/off-target toxicity). (c) Optimal clinical doses, as determined by both PS and TS, that balance efficacy and safety. STAR system prioritizes STAR class I drugs with high efficacy/high safety/high success rates, contrasting with the current emphasis on STAR class II/IV drugs with low efficacy/low safety/low success rates.
The Latest Manuscripts:
Can machine learning overcome the 95% failure rate and reality that only 30% of approved cancer drugs meaningfully extend patient survival? Duxin Sun, Christian Macedonia, Zhigang Chen, Sriram Chandrasekaran, Kayvan Najarian, Simon Zhou7, Tim Cernak, Vicki L. Ellingrod, H.V. Jagadish, Bernard Marini, Manjunath Pai, Angela Violi, Jason C. Rech, Shaomeng Wang, Yan Li1, Brian Athey, Gilbert S. Omenn. J Med Chem, 2024, 67, 16035
Why 90% of Clinical Drug Development Fails and How to Improve It? Duxin Sun, Wei Gao, Hongxiang Hu, Simon Zhou. Acta Pharmaceutic Sinica B, 2022, 12, 3049
Dual Targeting of STING and PI3Kγ Eliminates Regulatory B Cells to Overcome STING Resistance for Pancreatic Cancer Immunotherapy. Chengyi Li, Shuai Mao, Hongyi Zhao, Miao He, Meilin Wang, Zhongwei Liu, Hanning Wen, Zhixin Yu, Bo Wen, Mahamadou Djibo, Jinsong Tao, Yingzi Bu, Wei Gao, Duxin Sun (Under revision in Nature Cancer 2024). bioRxiv doi: https://doi.org/10.1101/2024.02.14.580378
A gastrointestinal (GI) locally-activating Janus Kinae (JAK) inhibitor to treat ulcerative colitis. Yingzi Bu, Mohamed Dit Mady Traore, Luchen Zhang, Lu Wang, Zhongwei Liu, Duxin Sun. J Biol Chem, 2023, 299: 105467
An oral tricyclic STING agonist suppresses tumor growth through remodeling of the immune microenvironment. Hong-Yi Zhao, Zhongwei Liu, Jinsong Tao, Shuai Mao, Meilin Wang, Miao He, Bo Wen, Wei Gao, Duxin Sun. Cell Chem Biol, 2025, 32, 280
2. Why most anticancer nanomedicines do not enhance clinical efficacy and how to improve it?
This project develops albumin based nanomedicines to enhance clinical efficacy of immuno-oncology drugs (STING agonists and PI3Kγ inhibitors) by targeting immune cells in the lymphatic system and tumors for cancer immunotherapy.
Anticancer nanomedicines hope to act like biological missiles targeting tumors to improve efficacy and minimizing toxicity in normal organs. However, despite their outstanding efficacy in preclinical animal cancer models, most anticancer nanomedicines have not demonstrated superior clinical efficacy, sparking decades long debate on current design nanomedicine design strategies.
We propose a drug/nanocarrier-specific nanomedicine design strategy to improve their clinical success: (1) Cancer-specific: identifying features of cancer types that can be used for targeted drug delivery; (2) Cell-specific: understanding the cell types to which drugs need to be delivered; (3) Drug-specific: identifying the intrinsic shortcomings of the delivered drugs that need to be overcome; and (4) Nanocarrier-specific: evaluating specific nanocarriers to overcome the specific limitations of the delivered drugs.
The Latest Manuscripts:
What Went Wrong with Anticancer Nanomedicine Design and How to Make It Right. Sun D, Zhou S, Gao W. ACS Nano. 2020 Oct 27;14(10):12281-12290.
Reappraisal of anticancer nanomedicine design criteria in three types of preclinical cancer models for better clinical translation. Luan X, Yuan H, Song Y, Hu H, Wen B, He M, Zhang H, Li Y, Li F, Shu P, Burnett JP, Truchan N, Palmisano M, Pai MP, Zhou S, Gao W, Sun D. Biomaterials. 2021 Aug;275:120910.
Albumin nanoparticle containing a PI3Kγ inhibitor and paclitaxel in combination with α-PD1 induces tumor remission of breast cancer in mice. Yudong Song, Luke Bugada, Ruiting Li, Hongxiang Hu, Luchen Zhang, Chengyi Li, Hebao Yuan, Krishani Rajanayake, Nathan Truchan, Fei Wen, Wei Gao, and Duxin Sun. Science Translational Medicine, 2022, 14, eabl3649
3. How to improve anticancer efficacy of neoantigen mRNA or peptide cancer vaccines?
This project develops SARS-CoV-2 B epitope-guided neoantigen peptide or mRNA cancer vaccine to enhance their anticancer efficacy by activating CD4/CD8 T cell immunity through B cell-mediated antigen presentation.
Cancer neoantigen vaccines typically rely on dendritic cell/macrophage-dependent antigen presentation to activate CD4/CD8 T cell antitumor immunity. Emerging evidence suggested B cell-mediated antigen presentation also plays a vital role in anticancer immunotherapy. It is not known whether incorporating B cell-mediated antigen presentation into current neoantigen vaccines could enhance their anticancer efficacy. We developed an innovative SARS-CoV-2 B Epitope-Guided peptide and mRNA neoantigen vaccines to enhance their efficacy in melanoma, pancreatic, and breast cancers, by more effectively activating CD4/CD8 T cell antitumor immunity through B cell mediated antigen presentation.
The Latest Manuscripts:
Antigen-Clustered Nanovaccine Achieves Long-Term Tumor Remission by Promoting B/CD 4 T Cell Crosstalk. Chengyi Li, Ryan Clauson, Luke F. Bugada, Bing He, Hongwei Chen, Hongxiang Hu, Polina Chuikov, Ke Fang, Brett D. Hill, Syed M. Rizvi, Yudong Song, Kai Sun, Daniel Huynh, Marilia Cascalho, Lana Garmire, Leo Yu Lei, Irina Grigorova, Fei Wen, Wei Gao, Duxin Sun. ACS Nano 2024, 18: 9584-9604
SARS-CoV-2 B Epitope-Guided Neoantigen NanoVaccines Enhance Tumor-Specific CD4/CD8 T Cell Immunity Through B Cell Antigen Presentation. Chengyi Li, Shuai Mao, Zera Montemayor, Mohamed Dit Mady Traore, Alejandra Duran, Fang Ke, Mahamadou Djibo, Hanning Wen, Wei Gao, Duxin Sun. ACS Nano, 2025, 19, 7038
4. What are the differences of microbiome, bile salts, and drug release in different regions of human GI tract?
This project investigates the differences of the microbiome, bile salts, and drug release among the human stomach, small intestine, and colon, as well as studies how these differences influence drug product development and disease states.
During oral drug product development, optimizing in vitro and in vivo drug release in the human gastrointestinal (GI) tract is crucial. Bile salts in the GI tract, which change under fasting and fed conditions, influence drug release, disease states, and the microbiome. Additionally, the human GI tract’s microbiome plays a role in regulating disease conditions and drug treatments. We directly measured drug release in various regions of the human GI tract (stomach, duodenum, jejunum, and ileum) for immediate-release, modified-release, and locally-acting drug products. We also compared the profiles of 15 bile salts in different regions of the human small intestine under fasting and fed conditions. Lastly, we examined the distinct microbiome profiles in different regions of the human small intestine and colon.
Spatial and Temporal Analysis of the Stomach and Small-Intestinal Microbiota in Fasted Healthy Humans. Seekatz AM, Schnizlein MK, Koenigsknecht MJ, Baker JR, Hasler WL, Bleske BE, Young VB, Sun D. mSphere. 2019 Mar 13;4(2):e00126-19.
In Vivo Dissolution and Systemic Absorption of Immediate Release Ibuprofen in Human Gastrointestinal Tract under Fed and Fasted Conditions. Koenigsknecht MJ, Baker JR, Wen B, Frances A, Zhang H, Yu A, Zhao T, Tsume Y, Pai MP, Bleske BE, Zhang X, Lionberger R, Lee A, Amidon GL, Hasler WL, Sun D. Mol Pharm. 2017 Dec 4;14(12):4295-4304.
Measurement of in vivo Gastrointestinal Release and Dissolution of Three Locally Acting Mesalamine Formulations in Regions of the Human Gastrointestinal Tract. Yu A, Baker JR, Fioritto AF, Wang Y, Luo R, Li S, Wen B, Bly M, Tsume Y, Koenigsknecht MJ, Zhang X, Lionberger R, Amidon GL, Hasler WL, Sun D. Mol Pharm. 2017 Feb 6;14(2):345-358.
Lab Members
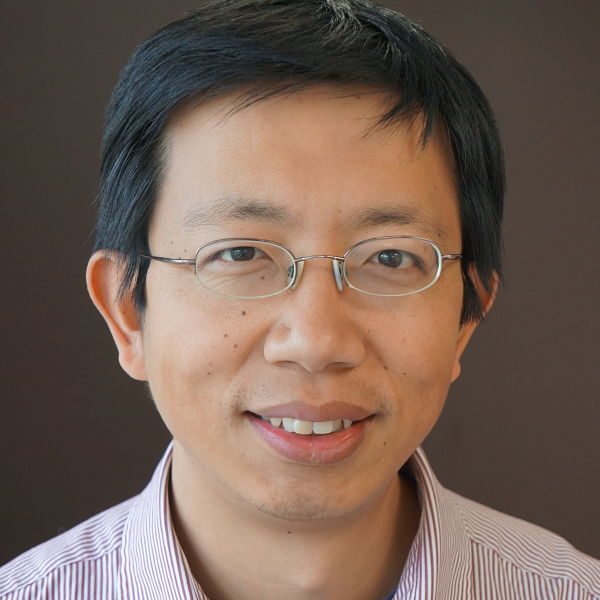
Bo Wen
Associate Research Scientist, Managing Director of PK Core
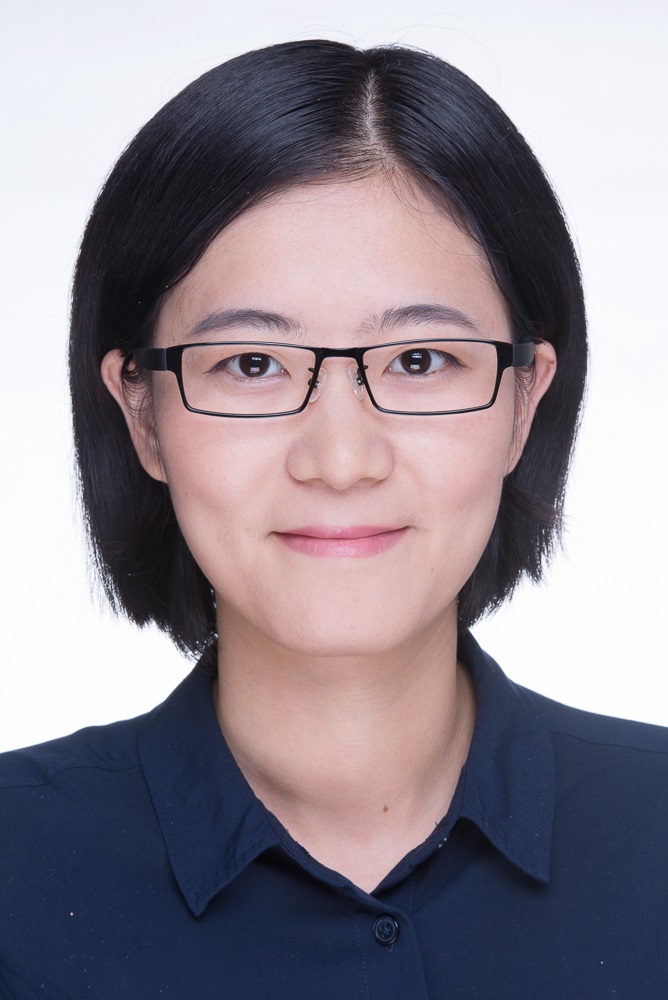
Fang Ke
Research Investigator
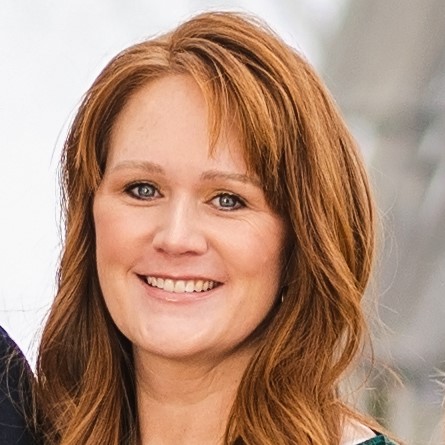
Erika Zucal
Administrative Specialist (Sun Lab and PK Core)
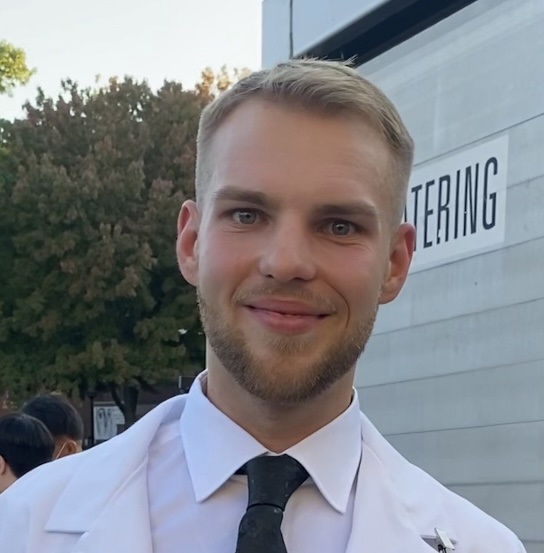
Zerick Dill
Lab Manager (Sun Lab and PK Core)
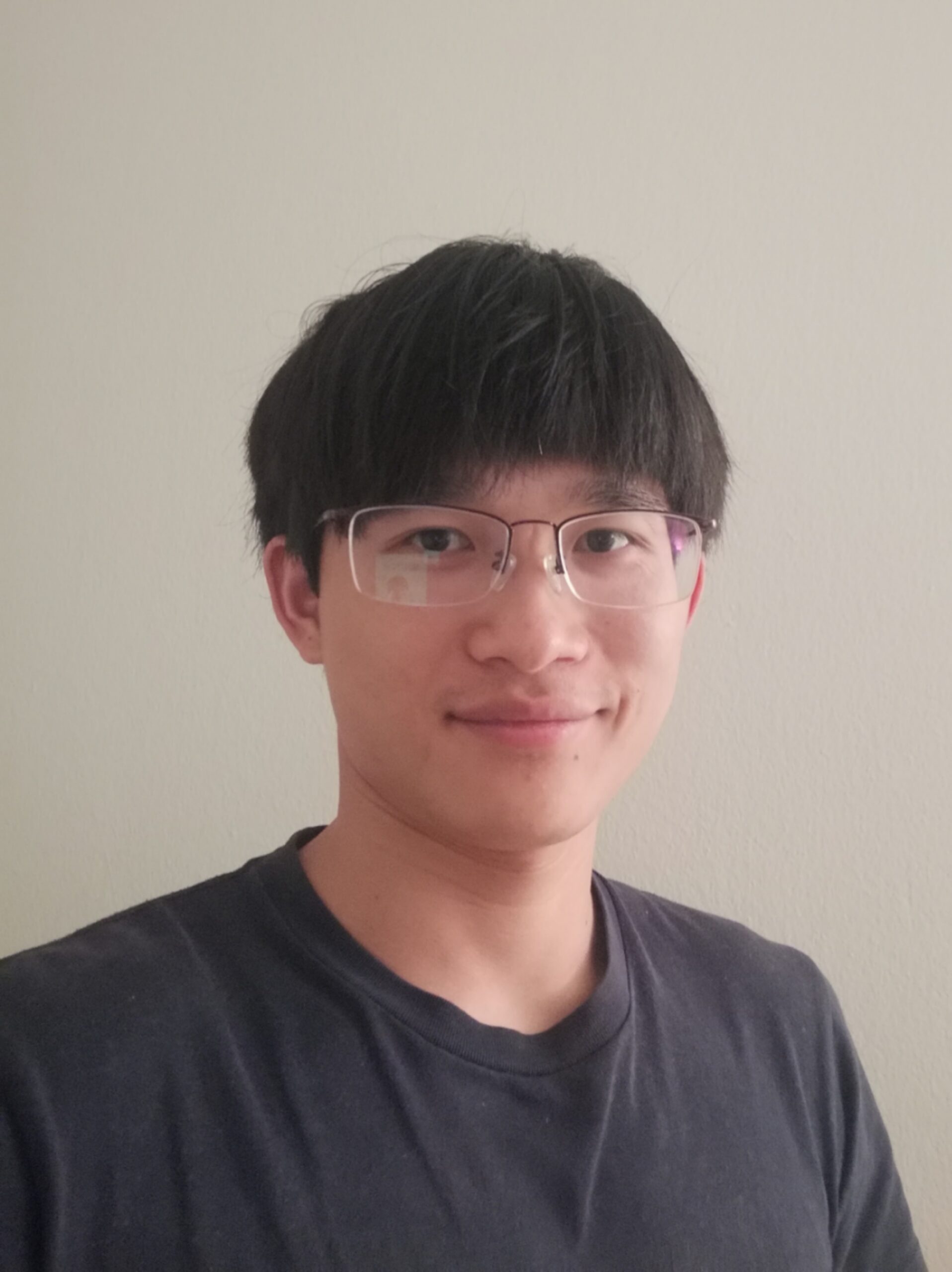
Chengyi Li
Research Fellow
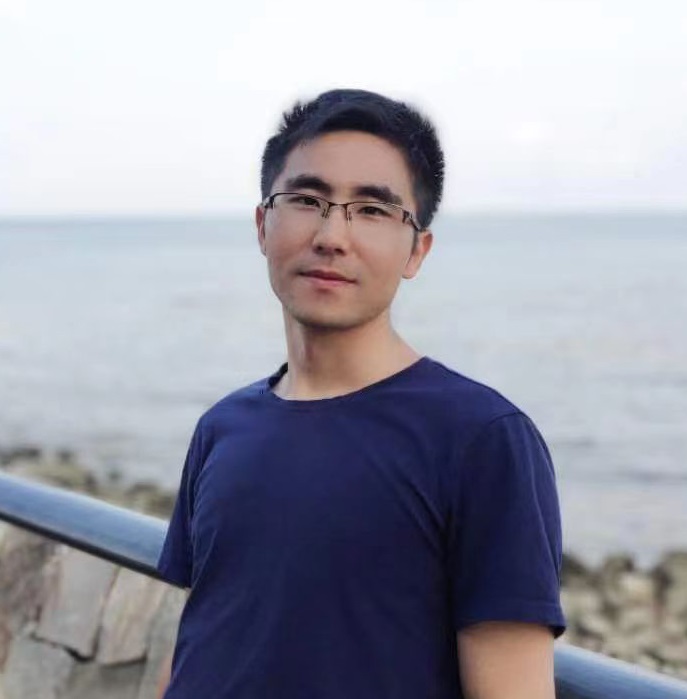
Jinsong Tao
Research Fellow
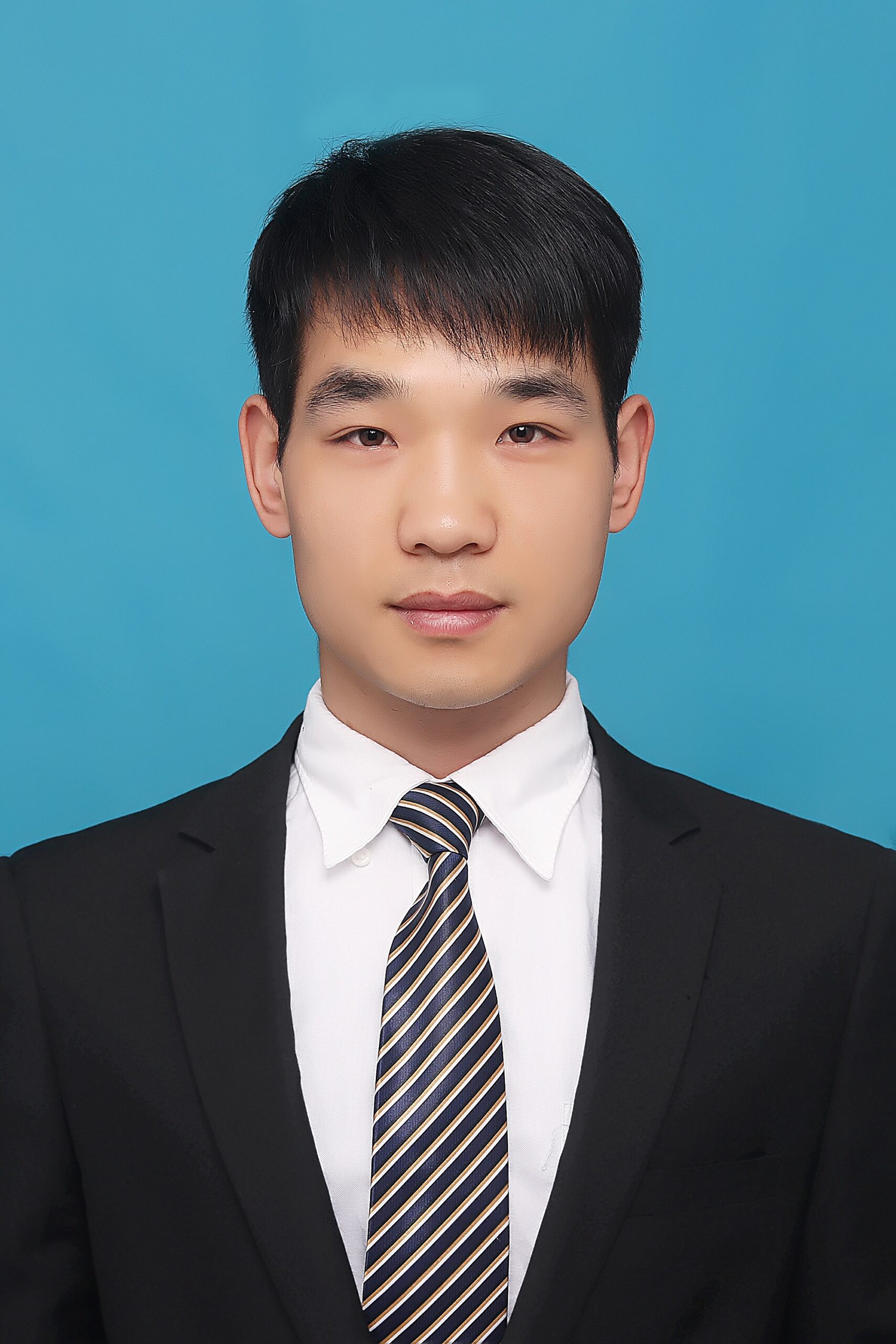
Hongyi Zhao
Research Fellow
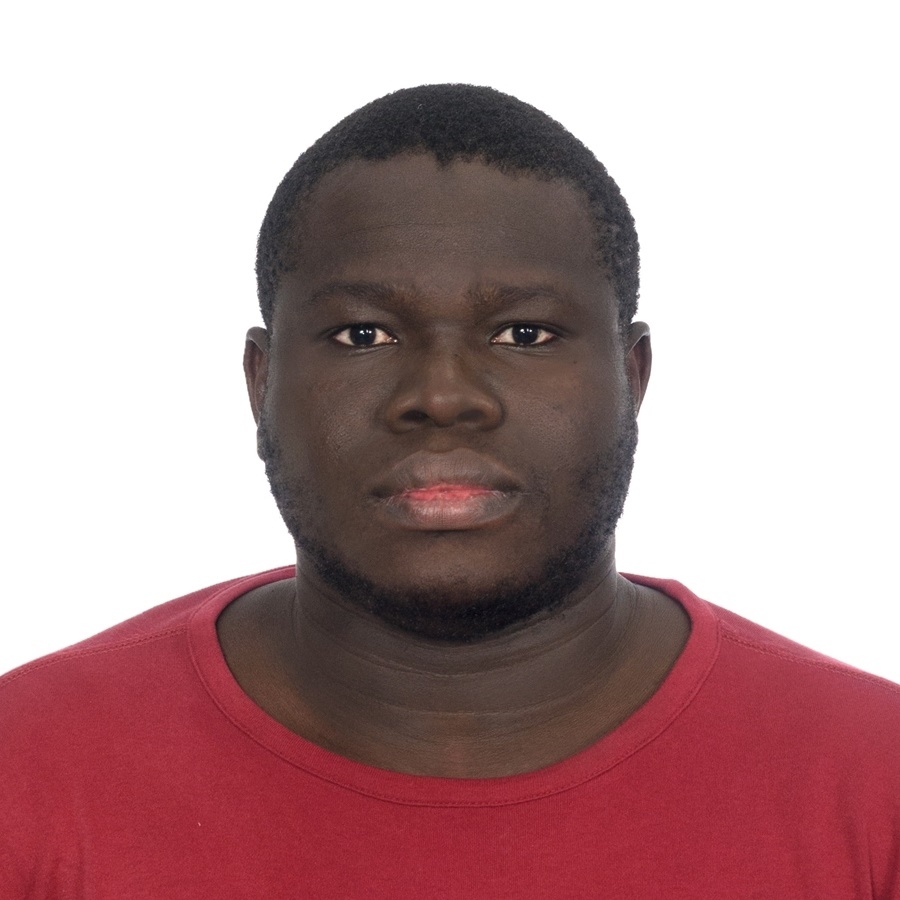
Djibo Mahamadou
Research Lab Specialist
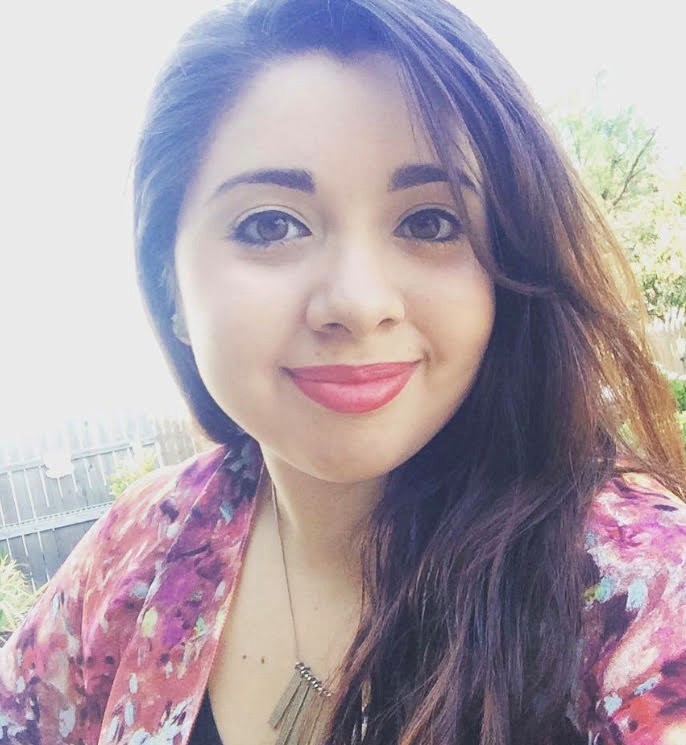
Zera Montemayor
Ph.D. Student
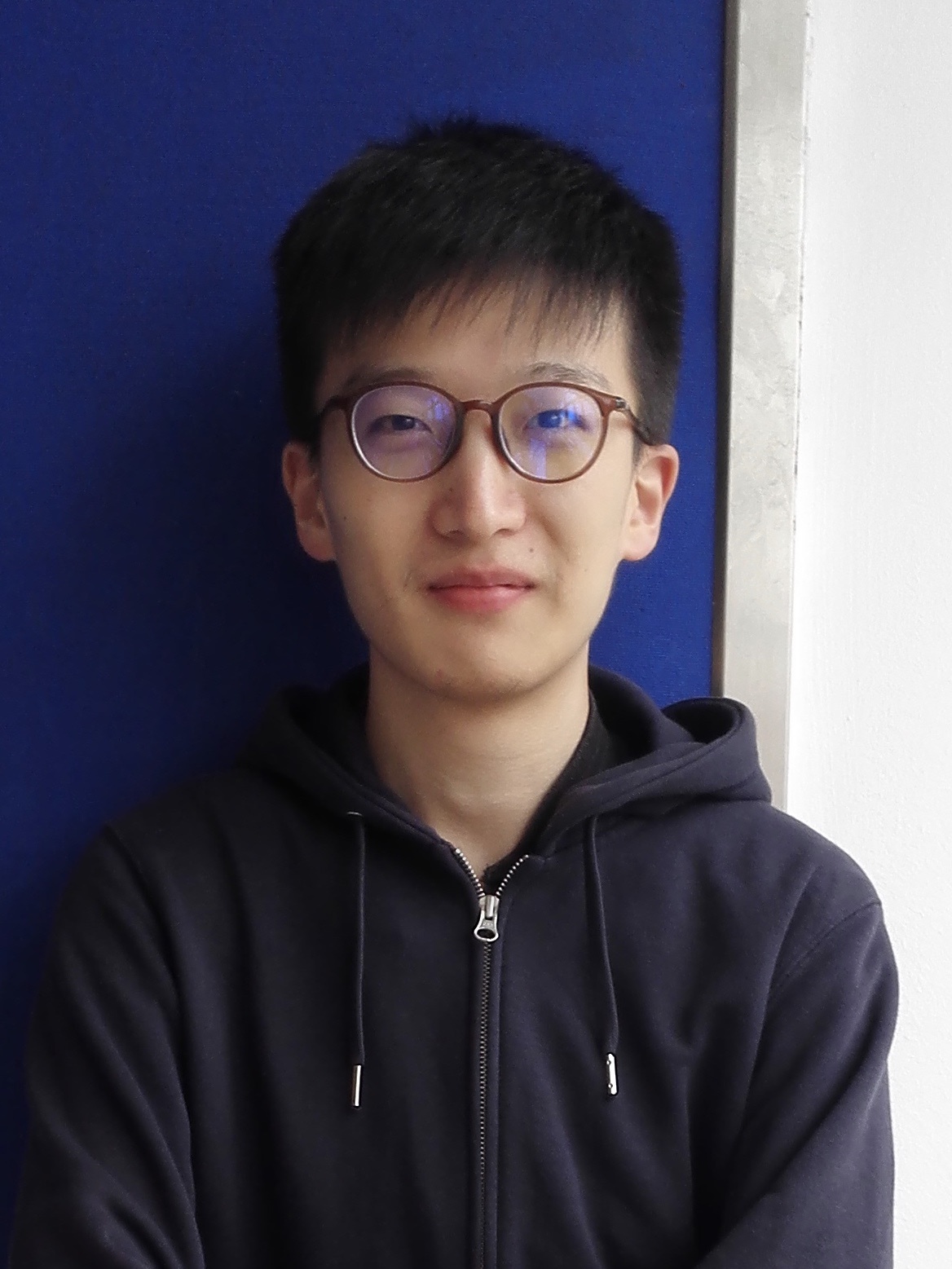
Luchen Zhang
Ph.D. Student
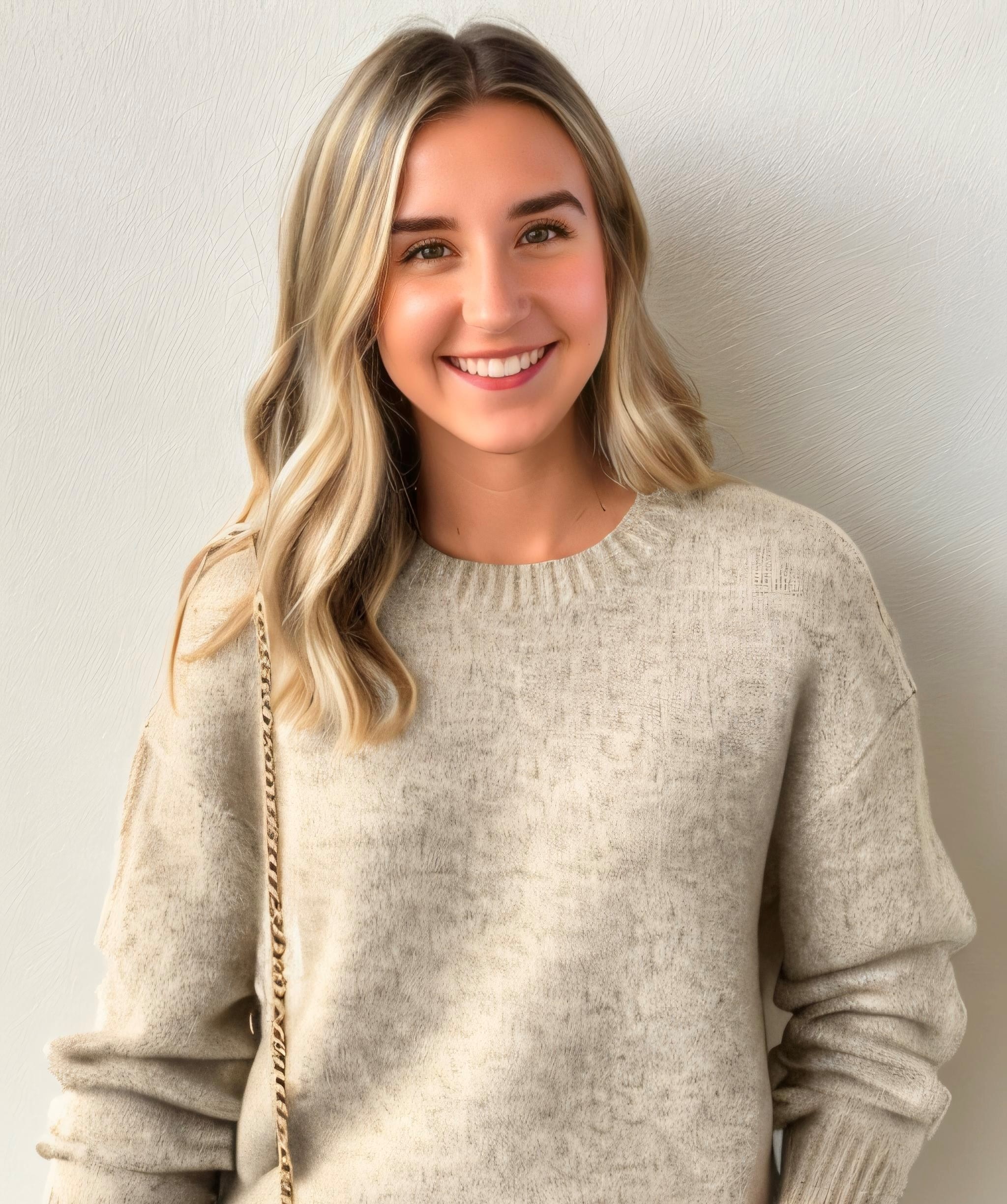
Natalie Jusko
Ph.D. Student
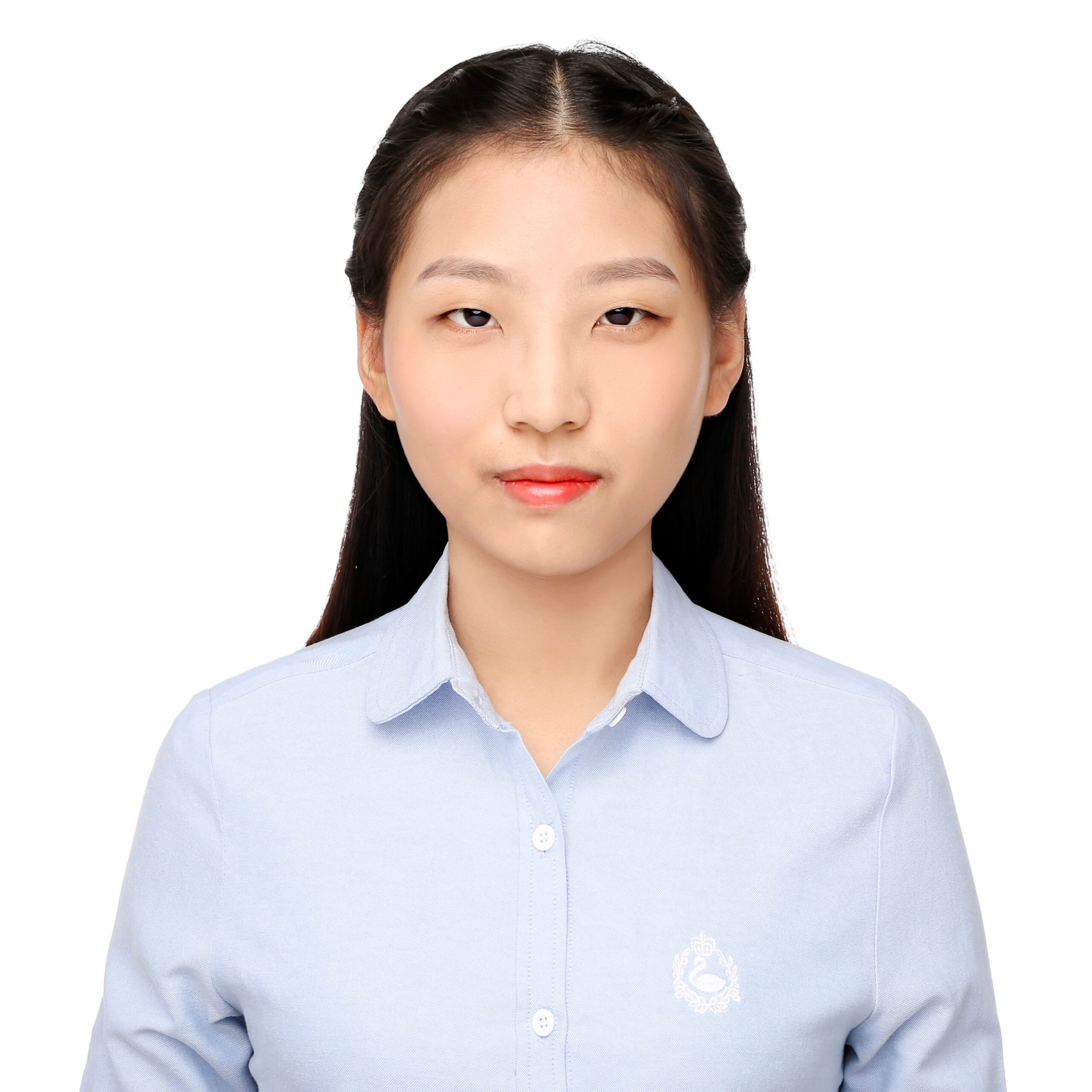
Hanning Wen
Ph.D. Student
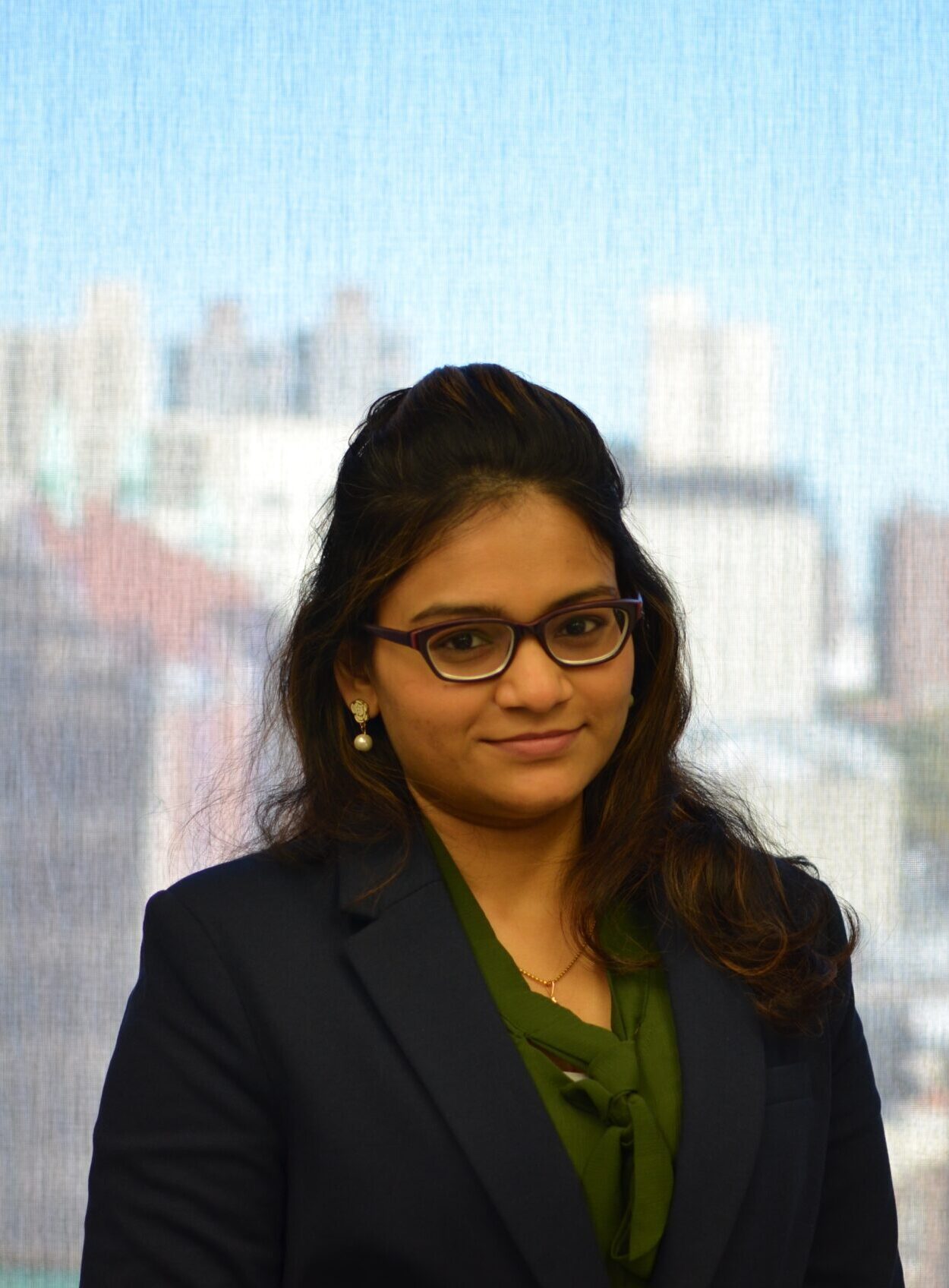
Neha Karekar
Ph.D. Student
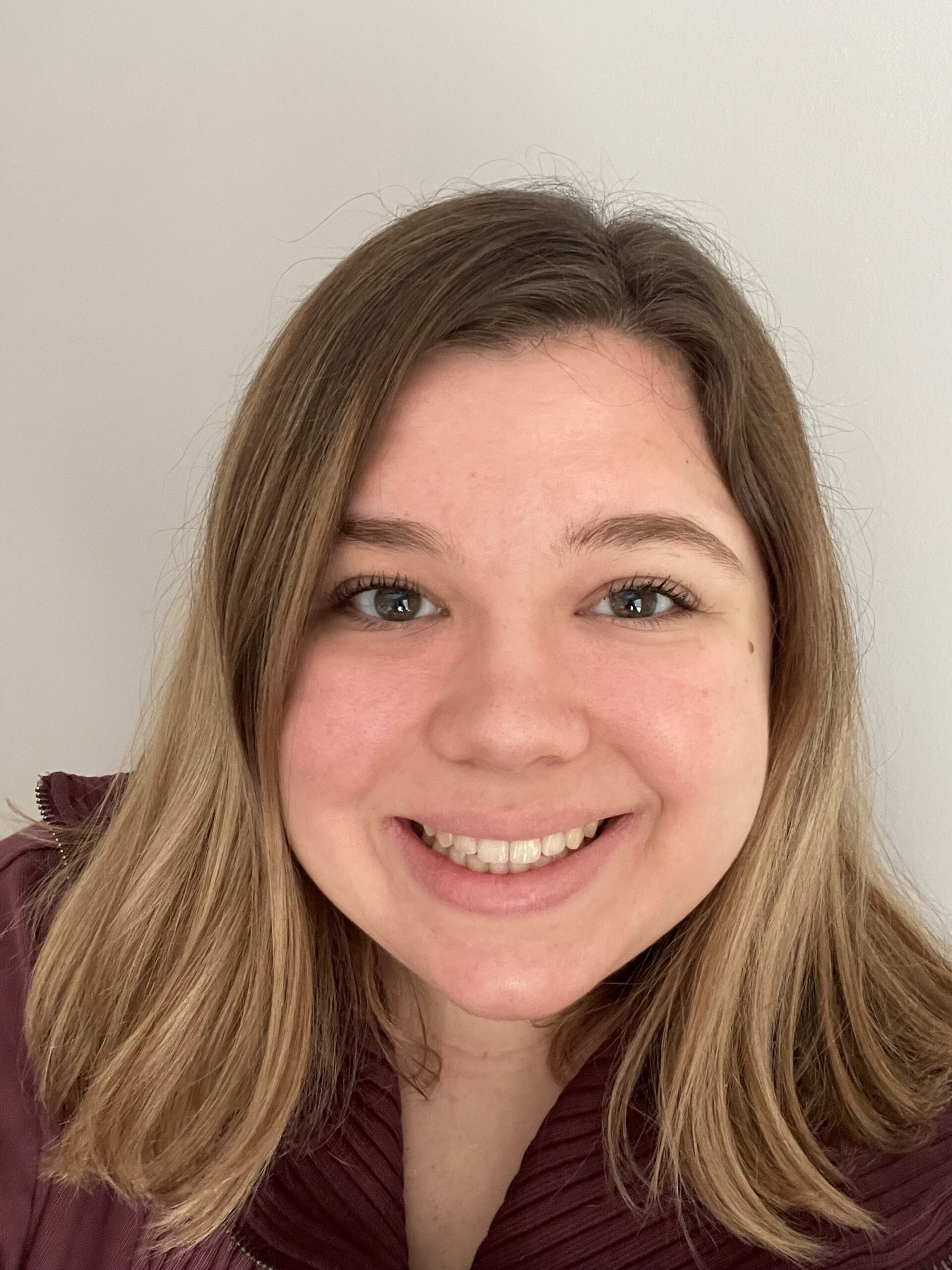
Hannah Myatt
Ph.D. Student
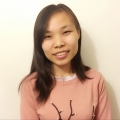
Lu Wang
Research Lab Specialist (PK Core)
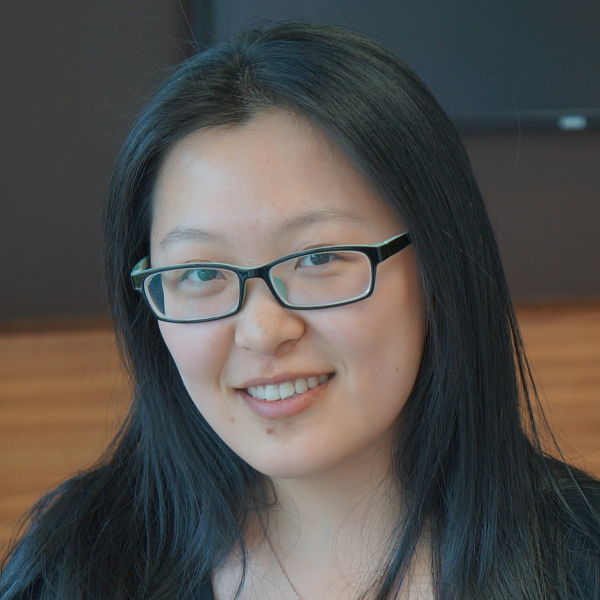
Miao He
Research Lab Specialist (PK Core)
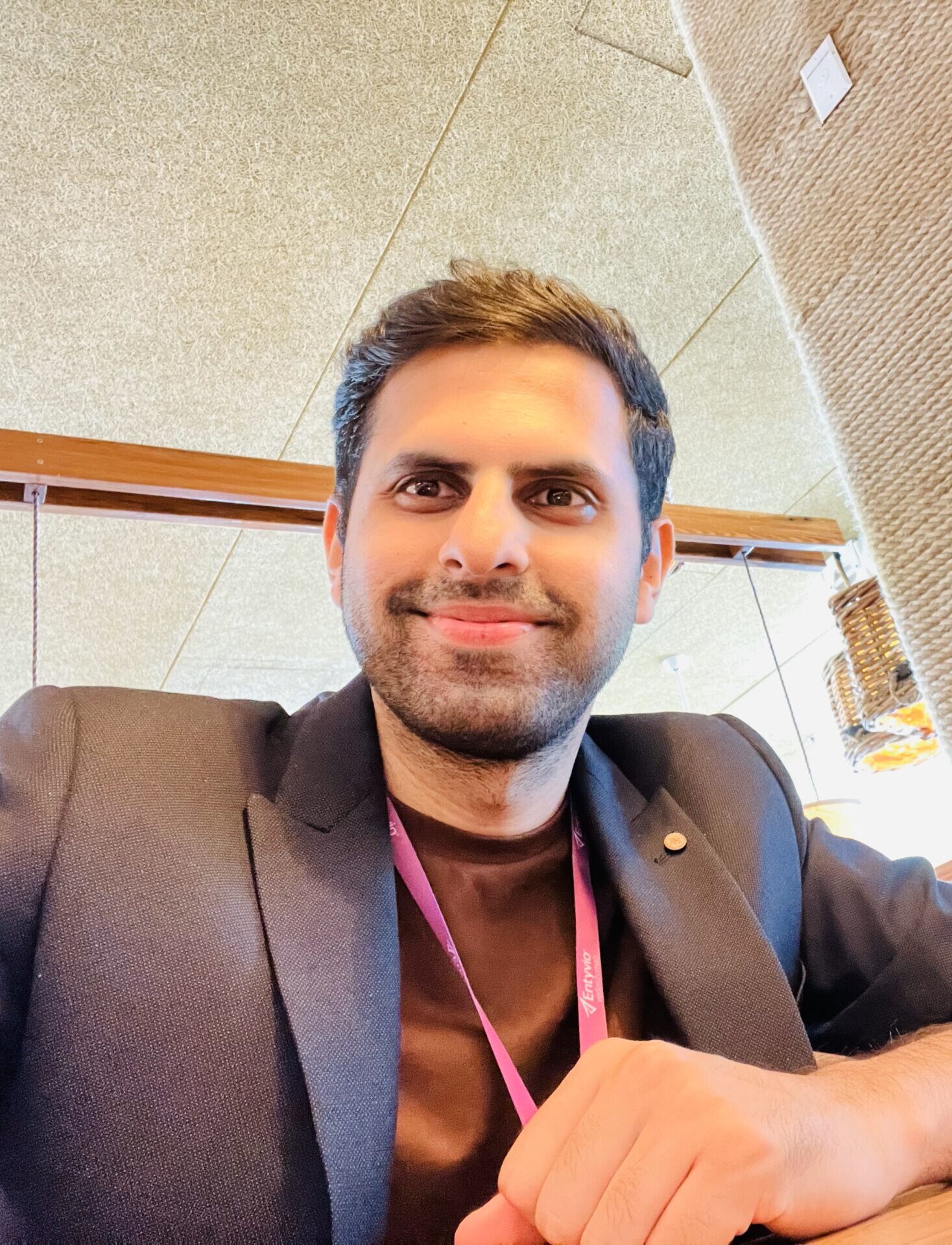
Arsalan Iqbal
Clinical Research Coordinator
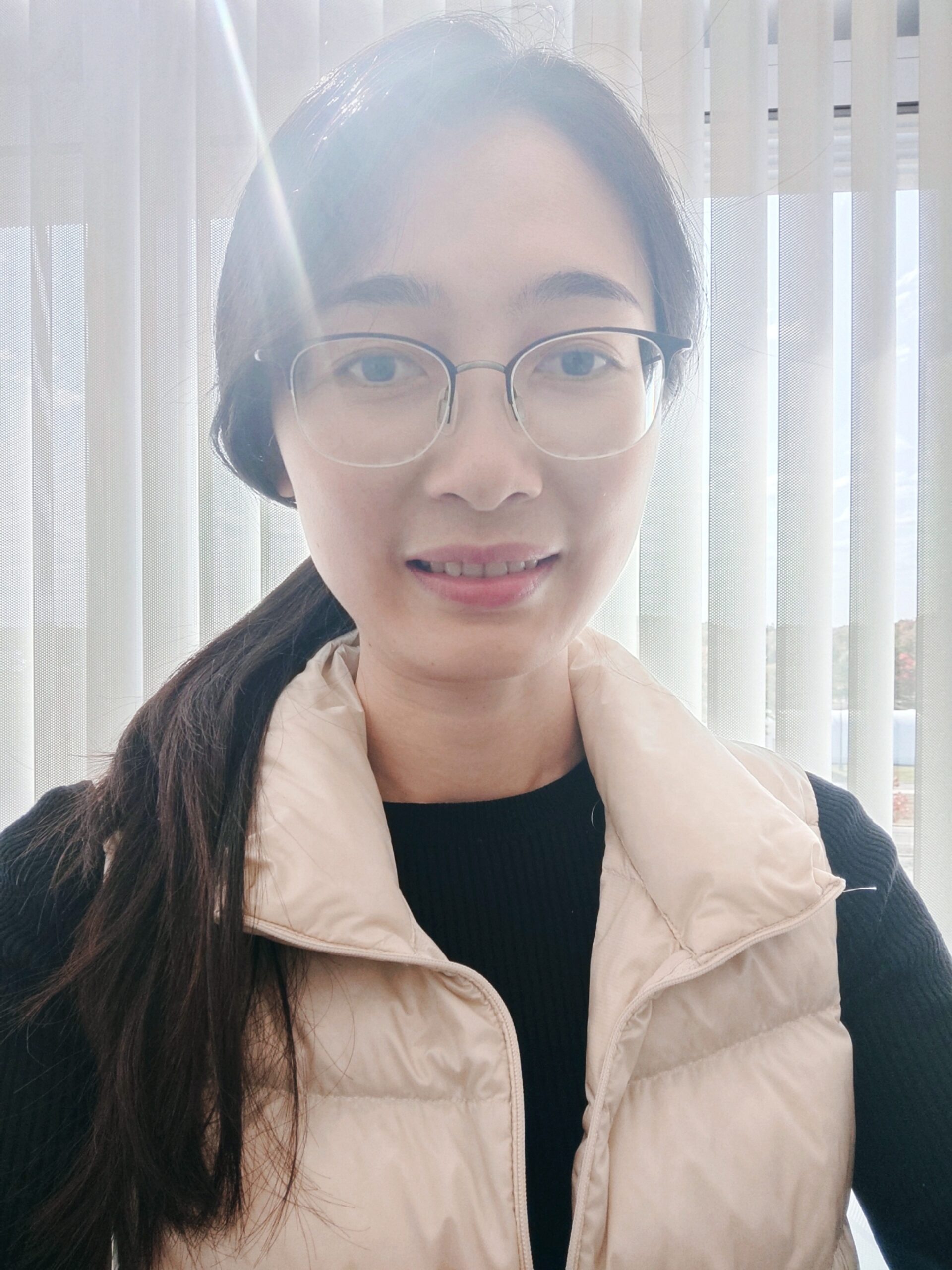
Qiuxia Li
Lab Technician (PK Core)
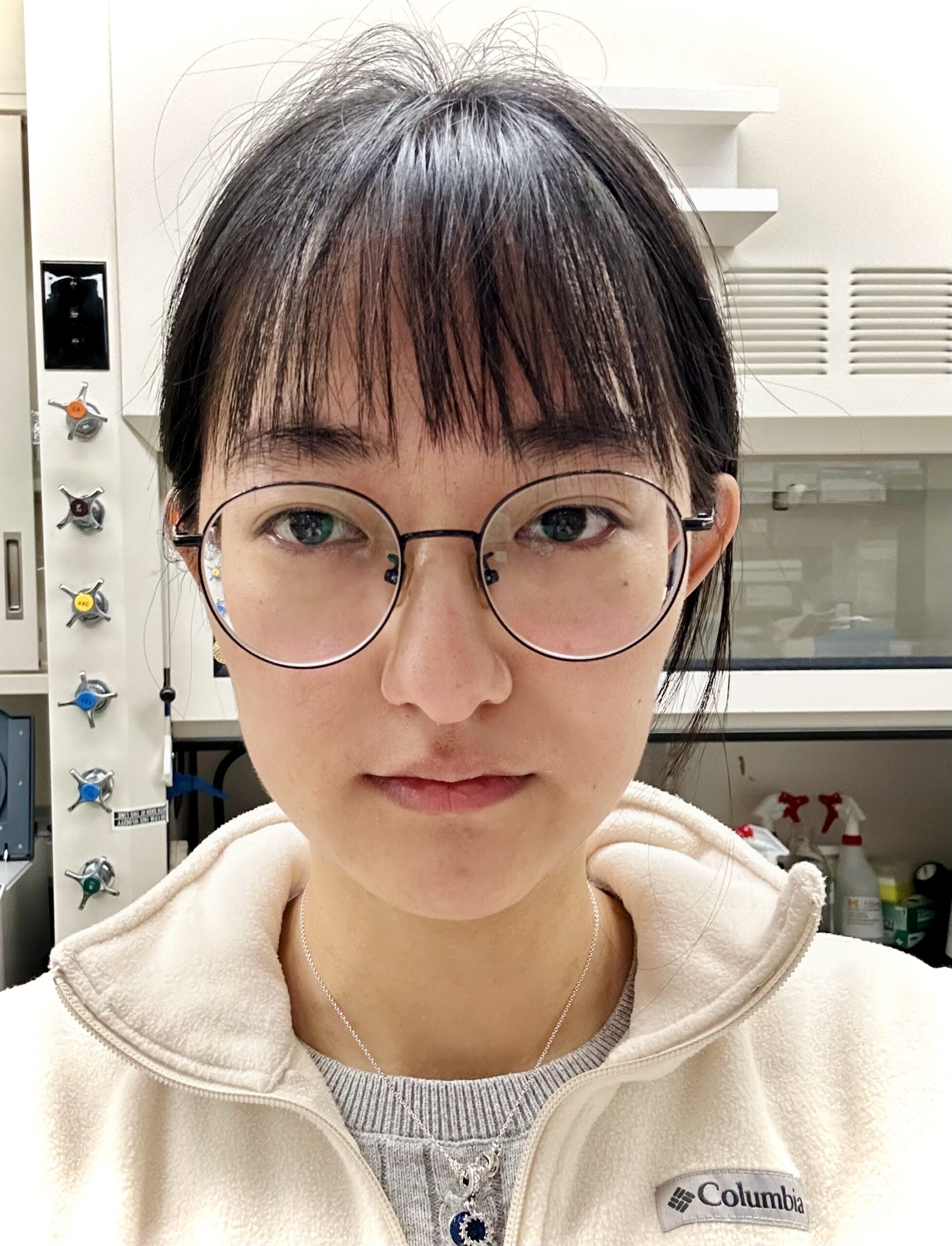
Shengnan Duan
Research Fellow (PK Core)
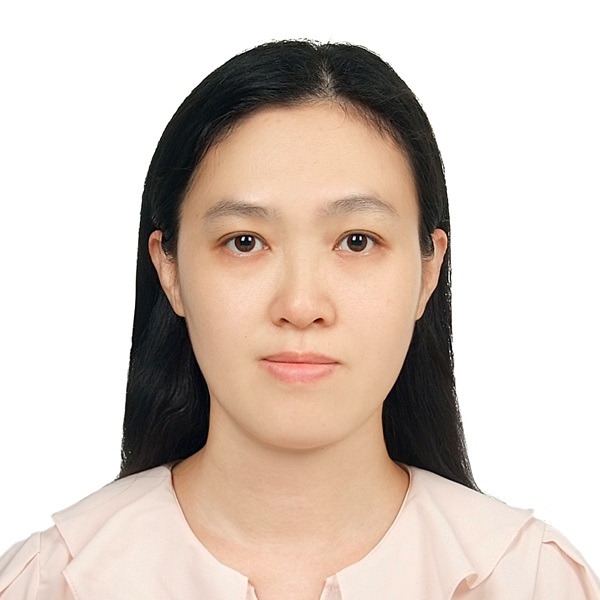
Tuyen Nguyen
Research Fellow
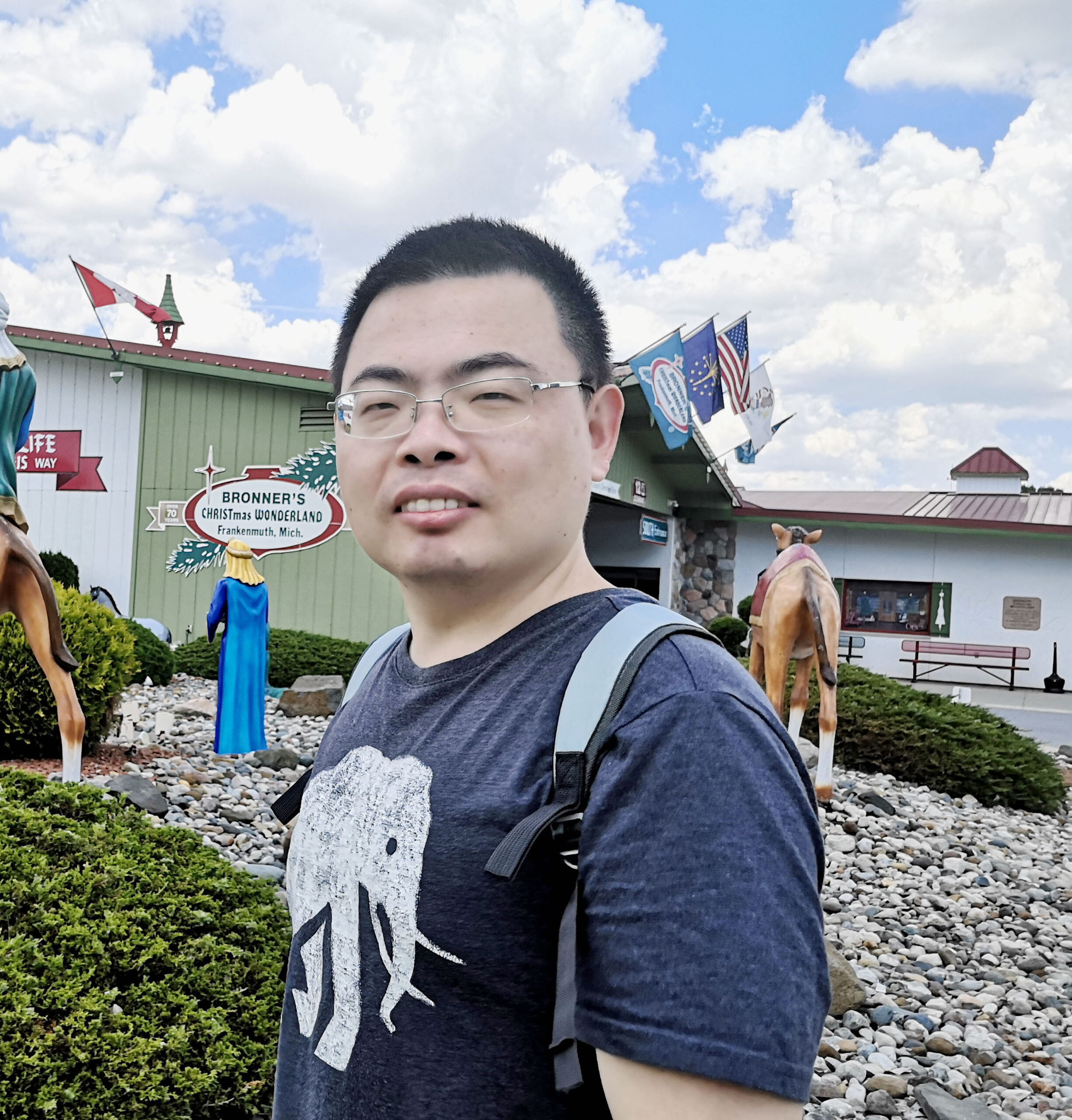
Meilin Wang
Research Lab Specialist (PK Core)
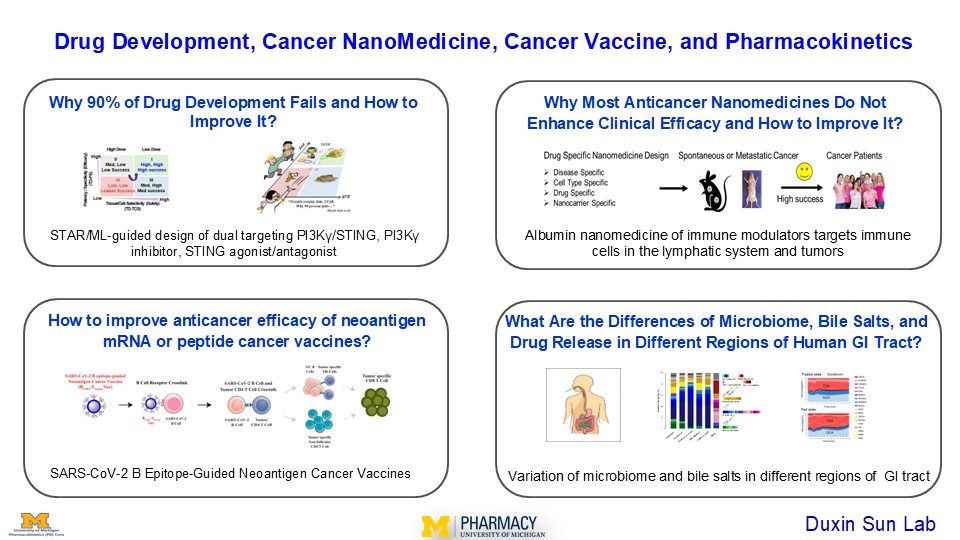